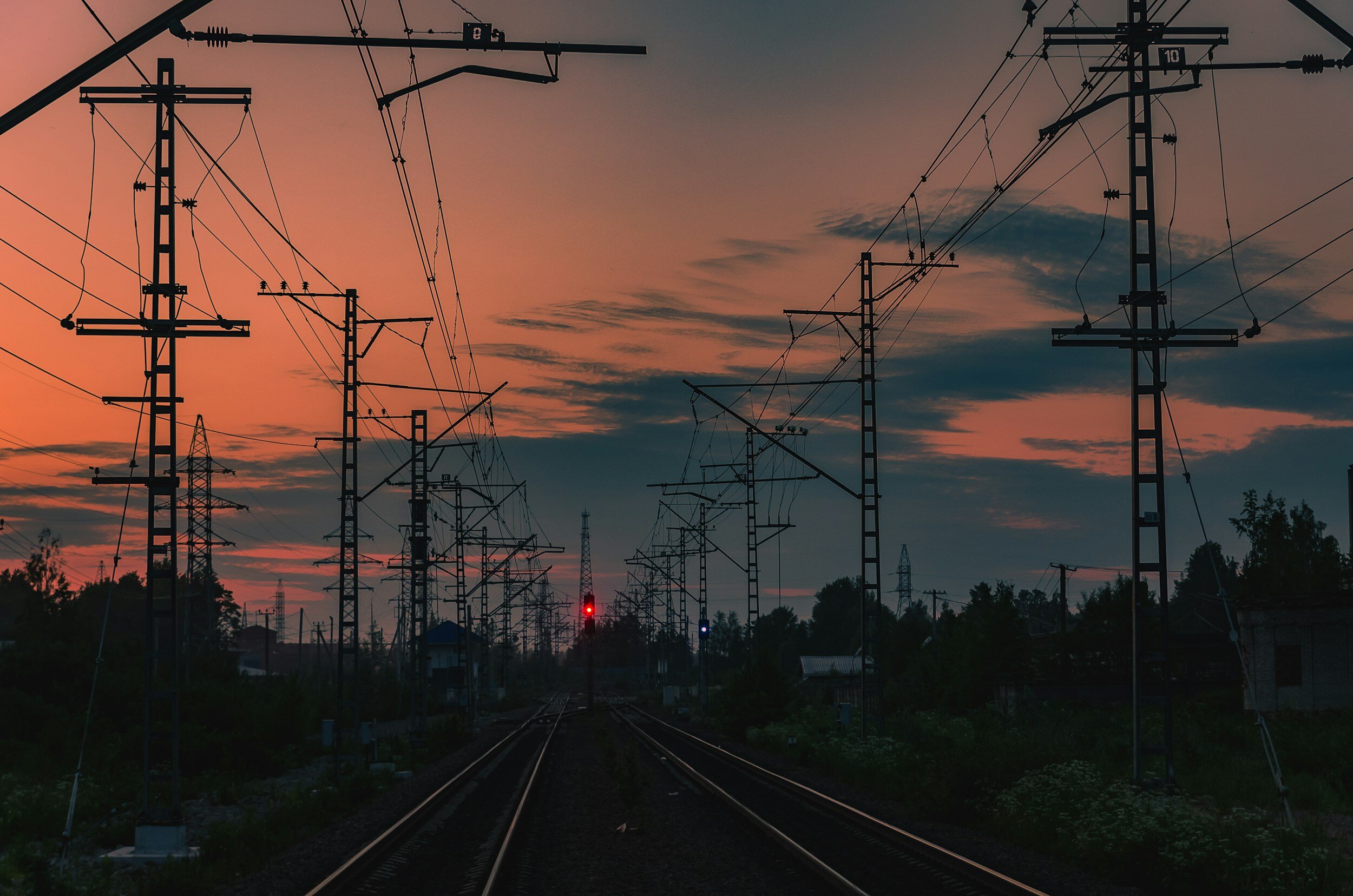
The Right Route to Rail Decarbonization is Electrification
Response to U.S. Dept. of Energy RFI — Progression to Net-Zero Emission Propulsion Technologies for the Rail Sector
While challenging, the transition to net-zero propulsion presents an excellent opportunity to modernize both passenger and freight rail transportation in the United States. The most common and cost-effective decarbonization technology is also the most common propulsion technology for trains: overhead traction power.
Across the world, the overwhelming majority of rail passengers—not to mention a growing majority of rail freight—travel on electrified infrastructure. This makes simple economic sense: electric trains are over 35% cheaper to operate than diesel trains [1], and in a Dutch benchmarking study they were found to have half the lifecycle cost of diesel trains in passenger service [2]. Perhaps even more importantly, electrification also offers strong operational and environmental benefits, as we explain in this document.
Several other forward-looking groups have also submitted a response to this RFI. We link to their reports in the Further reading section below.
Introduction
-
1. What is your view of zero-emission, or net-zero emission, rail propulsion technologies in the next 5 years? 10 years? 30 years? In your response, please include which rail propulsion technologies for line-haul and railyard operations do you see developing most promisingly. Please provide as many details as possible e.g., battery chemistry for batteries, charger type for electrification, fuel cell vs combustion, feedstock source, etc.
2. What efforts are you aware of to decarbonize rail transportation, including ways to reduce diesel fuel use? Are you aware of intermediate decarbonization milestones for rail transportation? Are you aware of longer term decarbonization goals for rail transportation? If so, describe how those goals might be met, including whether low-carbon biofuels will play a role.
3. What are the benefits and challenges of the various rail propulsion technologies as compared to the other alternatives? If possible, please provide a ranking of the alternative technologies starting with the most viable/promising option.
4. What obstacles to rail decarbonization is the industry facing? What plans can be put in place to overcome these challenges?
Most global railways are electrified—that is, equipped with either an overhead or trackside continuous power supply. Japan and most of Europe got an early start, electrifying their commuter lines by the early 20th century, followed by their main intercity routes from the 1950s onward. Electrification isn’t limited to passenger service, however: several countries which run American-style heavy freight trains have largely electrified their networks. Both the Russian and Ukrainian rail networks are about 50% electrified, with the most important passenger and freight lines wired. In the 21st century, China and India [3] have rapidly wired their tracks, with both countries running electrically-powered freight trains with double stacked containers under wires [4].
As all this shows, the case for the electrification of US railways is incredibly strong—strong enough that wiring the entire system may well prove to be fiscally and environmentally prudent. However, in line with others’ experience, the strongest case for electrification remains on passenger lines—and especially on urban commuter lines—and this is where any large-scale project should begin.
Nearly all existing and planned electrification consists of direct installation of overhead conductors above the tracks. Such equipment is called overhead line equipment (OLE), overhead contact systems (OCS), or overhead catenary wire [5]. Overhead lines overwhelmingly dominate existing and planned electrification even as some alternatives have cropped up, namely battery- and hydrogen-powered trains. Both of these alternatives have serious drawbacks compared to simple electrification, a fact born out by propulsion choice across the globe. Consider the case of Norway, the current world capital for battery-electric cars [6], where the commuter rail network for the 500,000-person Trøndelag region has installed wire to run hourly service. In the United States, by comparison, greater New York—with more than 20 million residents—still has large unelectrified sections on its commuter rail network, including ones that run half-hourly long trains with one-seat service to Manhattan.
While overhead wire costs capital dollars to install, it pays off in the overwhelming bulk of cases [7]. A recent study of passenger rail electrification by the Verband der Elektrotechnik, Elektronik und Informationstechnik (VDE), a German technical association that maintains electrical safety standards, found that—while the breakeven point depends on the size of the train, the terrain, and the difficulty of wiring—a line is typically best served by overhead wire if it runs at least two-car regional trains every half hour or more at the peak and best served by battery technology below that service level [8]. It found hydrogen to be completely cost-ineffective. In other words, the body of evidence sends a clear message that decarbonizing American rail propulsion requires a renewed emphasis on traditional overhead electric power as the dominant traction power solution.
For these reasons and more, electrification is the clear path forward for the US rail industry.
Benefits of conventional railway electrification
Rail systems benefit from OCS electrification for several reasons:
Reliability: Electrified systems benefit from at least a tenfold increase in reliability over diesel trains [9]. Perhaps counterintuitively, electric trains are so much simpler to operate and maintain than the diesel trains that dominate US railroads that their cost savings more than offset the maintenance cost of the wire they use to operate. Electric trains contain far fewer moving parts than diesel ones. In most cases, the electric motors—one per axle—are the only moving parts. In contrast, diesel trains rely on an internal combustion engine with pistons and a crankshaft to turn a generator that powers the same electric motors attached to the wheels, a far more complicated arrangement with much greater wear due to vibration from the engine.
Acceleration: At typical suburban rail speeds, modern self-propelled electric trains —called electric multiple units (EMUs)—save around 30 seconds of travel time per stop over diesel train sets of the same passenger capacity. This not only speeds service but also permits planners to add more stops to schedules with minimal impact on overall travel time, increasing the usefulness and legibility of service. In the Bay Area, for example, Caltrain plans to turn many limited-stop trips into local trips when it converts to EMUs; local trains from San Francisco to San Jose will be 25% faster than today [10].
Power-to-weight ratio: Electric trains that draw power from an overhead or trackside power supply are much lighter, and can draw more power, than those that carry a diesel engine, hydrogen fuel cells, or batteries. This is one reason electric trains accelerate faster. Moreover, as they can draw surge power for short times, electric trains can climb steeper grades than diesels can manage. It is telling that mountainous Switzerland has a fully electrified system, and that the longest continuous section of electrified track installed in the United States was the Milwaukee Road’s Pacific Extension, which crossed the Rockies and Cascades.
Lack of pollution: Electric locomotives do not emit combustion pollutants, so nearby communities benefit from cleaner air. The same is true of travel through tunnels, which by their nature limit ventilation. Right now, the performance of diesel trains through such key tunnels as the Stevens Pass is limited by the exhaust capacity of ventilation systems, creating choke points on important transcontinental routes. In an urban context, many commuter lines that pass through dense neighborhoods with high asthma levels still run polluting diesel locomotives, for example Boston’s Fairmount Line [11].
Higher ridership: All of the benefits mentioned above don’t merely reduce long-term operating costs and environmental impact, they also provide a better experience for passengers. The result is increased ridership, a phenomenon that British proponents of rail electrification call the sparks effect [12].
All of these benefits scale with rail traffic. For example, consider passenger systems that run a large amount of peak service. This requires a large number of train sets. Not only do EMUs cost less to operate, but because they are faster and more reliable, fewer train sets are required to run a given service level. As a result, electrification brings major lifecycle cost savings. The same phenomenon applies to air pollution. Since pollution is created by each and every moving train, electrifying busy main lines can massively reduce pollution. This means that the returns on electrification are the highest on the most intensively-used lines. This is why typically electrification programs begin with commuter lines, as they did in early-20th century America with New York- and Philadelphia-area lines.
Nonetheless, the American rail network has so little electrification and so much rail traffic that there is a strong case for electrifying substantial parts or even the entire national network of passenger and Class I freight rail lines.
Decarbonizing passenger rail should start with the electrification of urban passenger lines
Many unelectrified US commuter rail lines already run enough service to justify the cost of catenary electrification. Service on almost every American commuter rail line exceeds the VDE threshold for overhead wire of a two-car train every half-hour [13].
This is true, for example, of most of the commuter rail lines in the greater Boston area operated by the MBTA. Most of these lines already run six- to eight-car trains two to four times per hour in the peak direction. What’s more, the MBTA is now seeking to run service even more frequently, as MBTA commuter rail has already regained over 90% of its pre-pandemic ridership [14], and expects to exceed it in the near future.
In greater New York, ETA’s home, there exist a number of high ridership lines which nonetheless lack electrification, including the Upper Hudson Line of Metro-North and the Raritan Valley Line of New Jersey Transit. Both services run about five peak trains per hour, all six to eight cars long, which makes for a benefit-cost ratio of electrification around 10 [15]. The peak frequency on other unelectrified lines such as the Long Island Rail Road (LIRR) Montauk Branch between Babylon and Speonk, the New Jersey Transit Montclair-Boonton Line, and the Metro-North Danbury Branch average about an eight-car train every half hour. While these are far from the strongest diesel branches and thus are currently considered low priorities for electrification, adding wires would still be the most effective way to increase the amount and quality of passenger service while also decarbonizing.
Conventional electrification for freight rail
-
9. What type of service testing, or derisking, of these propulsion technologies do you think are necessary for each alternative rail propulsion technology?
14. In your opinion, how do certain technologies (e.g. battery) compare for different use cases (e.g. line haul, switching)?
15. In your opinion, what percentage of overall locomotives could reasonably be expected to be zero-emission locomotives between now and 2050? How do you think production might scale up over time?
16. How do you think power needs should be estimated for the rail industry over time? E.g. number of locomotives or switchers?
17. What do you think should be the estimated global market size for net-zero emission locomotives or retrofitting technologies?
Conventional electrification is a proven, low-risk technology for freight rail: indeed, it is the global standard for train propulsion. As early as 2010, a majority of Indian rail freight traveled on electric trains [16], at which point 30% of its network was electrified. Since then, it has completed OCS over nearly the entire country’s network. While some US freight railroads claim that it is not possible to operate double-stacked freight under OCS, both China and India already run extensive double-stacked electric freight under wires. In the US, CSX runs double-stack freight powered by diesel locomotives underneath traction wire on the SEPTA West Trenton Line [17]. While the US has a larger legacy network than India or China, comparable in size to that of Europe, traffic tends to concentrate on a few main lines; thus an electrification program that captures most US ton-miles would require a relatively limited scope. Studies conducted in support of the 1980s BC Rail Tumbler Ridge project found that, at the time, one electric locomotive was the equivalent of two diesels [18]. If this ratio still holds true today, the estimated 25,000 locomotives the Class I railroads use could potentially be replaced with only 12,500 electric units.
The cost of rail electrification is significant but affordable. One estimate pegs the cost of wiring a typical Class I line around $2 million per route mile [19]. Entire main line segments can likely be wired between major crew change points for hundreds of millions of dollars each, which is comparable in cost to many transit projects that have recently been awarded federal funding. Replacement of all the electrification infrastructure on the approach to Paris’s Gare d’Austerlitz is to be completed shortly after 6 years of exclusively nighttime work, auguring well for the prospect of installing OCS with tolerable disruption [20].
Thanks to the worldwide popularity of electric traction, widespread production facilities already exist for locomotive and EMU construction. A Siemens Mobility press release on a €3 billion Indian locomotive order says 1,200 locomotives are to be delivered over an eleven-year period [21]. It is important to note that Siemens plans to fulfill the aforementioned order from one factory in India and already operates several more around the world, including in the United States. While the North American freight rail fleet is large, containing about 40,000 locomotives [22], a combination of existing manufacturing capacity and capacity that decarbonization investment would spur should be able to turn over a large fraction of the North American locomotive fleet within several years.
The VDE study looks at the use case of regional passenger rail, featuring low speeds, short multiple-unit trains, and frequent stops. The frequent stops encourage electrification over diesel, due to the aforementioned performance boost. In contrast, freight trains are very long–which makes electrification more valuable. However, when comparing different electrification technologies, the main tradeoff between OCS and battery technology is that OCS requires investment in fixed infrastructure whereas battery technology incurs higher vehicle costs; thus, even though the analysis studies multiple-units and not freight locomotives, its conclusions are likely to hold for line haul freight and not just regional passenger rail. For more on battery-electric technology, see the section below.
Battery trains
-
8. What infrastructure is required to support promising alternative rail propulsion technology? Are there specific routes, railyards, or network segments that would be a good candidate for alternative propulsion technologies (e.g., catenary, hydrogen fuel cells, or batteries)?
While battery freight locomotive technology has made some strides, the most powerful units can still only supplement diesel propulsion over relatively short distances [23]. Current battery energy density reaches less than 10% that of diesel fuel. That is expected to increase to 15% diesel’s energy density by 2035, which would still imply bulky and heavy onboard energy storage [24]. Battery vehicle range suffers in cold weather [25]. Due to that drawback, battery freight locomotives are likely to find their greatest use in first- and last-mile solutions such as yard and facility switching, and line-haul application almost certainly favors traditional OCS.
Meanwhile, precisely because freight trains outside North America largely run under wire, the market for battery locomotives is undeveloped. In contrast, electric locomotives powered by OCS have many vendors capable of fulfilling large orders [26].
The battery EMU (BEMU) market is more developed, largely for European regional lines with so little traffic that wiring them may not be economically justified. As such, some American passenger railroads, such as Metra and the MBTA, have expressed interest in battery-powered trains as an alternative to OCS, citing the lower cost of fixed infrastructure and challenges such as clearance. Many railroads around the world have experimented with battery trains and found little savings over traditional electric propulsion. Despite ongoing predictions of large advances, battery propulsion is still a nascent technology that has suffered notable setbacks. Planned battery train deployments in the Netherlands [27], on Long Island [28], and in New Jersey [29] have failed to materialize. BEMUs recently purchased by Liverpool’s Merseyrail have been fraught with mechanical problems [30]. BEMU deployment on high-ridership corridors is inappropriate for several reasons.
First, BEMUs have around a 100% cost premium over conventional EMUs [31]. Because costs increase with frequency and number of cars per set, and because train sets are recurring investments, this would drive up costs relative to conventional OCS methods. Moreover, charging time drastically lengthens the amount of time that trains lay idle, requiring more trains to be purchased for a given service level than with OCS. For this reason, active BEMU plans in Europe and Asia are generally limited to low-frequency lines running no more than three-car trains.
Second, most BEMU projects save little infrastructure capital cost over traditional OCS. To avoid significant charging time as mentioned above, BEMUs nearly invariably require extensive wired track to charge en route. Worse, the substations must supply high power intermittently to charge the batteries, increasing their cost and negative impact on the grid. Most of the recently deployed BEMUs charge partially from preexisting and/or partially extended wire and run through onto unelectrified, lower-ridership segments. For instance, Baden-Württemburg recently chose battery propulsion to extend electrification on its mostly-electrified system and plans to add OCS to some currently unequipped track [32]. This is likewise the MBTA’s current plan, and it requires approximately 30% of the Newburyport/Rockport Line’s full track mileage to be electrified.
Third, battery trains are likely to be slower and less reliable than their EMU counterparts, reducing ticket revenue and mode share. The combination of weather and limited battery service life is likely to introduce significant variability in charging times at endpoints. Furthermore, on a recently debuted BEMU, engineers had to eliminate traction motors from one of four bogies to fit the batteries, cutting power output by 25% [33]. On that train set, the power reduction adds 15 to 20 seconds of runtime per stop.
Hydrogen trains
-
8. What infrastructure is required to support promising alternative rail propulsion technology? Are there specific routes, railyards, or network segments that would be a good candidate for alternative propulsion technologies (e.g., catenary, hydrogen fuel cells, or batteries)?
Hydrogen-powered trains, another occasionally proposed alternative to OCS, fare worse against it than battery stock, and an overall energy efficiency of less than 40% (compared to about 90% of conventional OCS electric trains) [34]. Baden-Württemberg found hydrogen solutions would cost nearly double either battery trains or a fully wired system [35]. An analysis by Deutsche Bahn found that per seat-mile, hydrogen or battery propulsion would consume double the energy of overhead electrification [36]. Moreover, the water required to produce hydrogen for propulsion is likely to strain supply in dry areas [37].
By contrast, there is little reason to suspect that electrified rail would strain the American power grid. Estimates peg the Auckland commuter rail network’s total demand at under 1% of the electrical system’s capacity [38].
Cost control
-
13. Are you aware of any goals for Total Cost of Ownership (TCO) willingness to pay for advanced technologies? Recognizing that DOE and industry are driving to cost parity with diesel in the long term, what do you think the goals should be regarding reasonable extra costs over the diesel baseline in the near term?
US electrification projects have cost more than overseas ones, largely due to correctable factors, and DOE should prioritize increased efficiency. The Caltrain electrification project cost $14 million per mile compared to $2-3 million per mile elsewhere, largely because it diverged from project management best practices [39]. Excessive numbers of masts per mile contributed significantly. Moreover, the agency failed to coordinate signaling system and traction power system design and installation, leading to repeat work. Contractors reported long processes for adjusting pole locations based on deviations between planned and real-world ground conditions.
Lessons from the Great Western Electrification Programme [40] in the UK point to further cost savings on future American OCS projects. An overly conservative approach resulted in piles up to 30 to 50 feet deep, severely complicating their installation, triggering additional right-of-way requirements, and often needing redesign. On other UK [41] and European lines, poles are located directly beside the track, and piles reach 15 feet into the ground. It is estimated that use of more permissive standards saved over $300,000 per single-track mile on Scotland OCS installation [42]. For mast foundations, test holes add track outage time and increase the final required pile depth [43]. Use of ground penetrating radar instead of test holes for foundations has the potential to reduce cost and duration of electrification projects [44]. Lightweight masts made from composite materials, being tested in the UK, also offer potential for lower foundation costs [45].
Moreover, American standards currently require excessive clearances above the train [46][47]. While the tallest US freight cars reach about 20' 3" above the rail and many overhead structures afford little more than 1' to the car roof, California standards effectively prescribe a full 27' between bridges and rails on electrified railways—including those where no tall freight cars would realistically run. Moreover, it has been aiming for a pantograph envelope larger than any in use on any high speed rail line to date [48] and designing around a typical wire height around 8" higher than seen on European systems [49].
By contrast, other parts of the world have developed and validated strategies that reduce the worst-case clearances, saving large amounts of money that would otherwise be spent on rebuilding civil structures [50]. For example, pantograph horns on many European and UK trains are non-conducting [51]. Where bridges impose clearance challenges, Denmark and the UK have used surge arrestors, wire covers, and insulated paint [52] to reduce the clearance from overhead wire to structure from 10.6" to 5.9" in certain cases. Extensive testing has suggested even further clearance reductions from the wire to both the train roof and overhead structures are possible [53]. By contrast, California standards call for several feet of clear space to the side and above the pantograph [54]. By one estimate, thoughtful application of clearance reduction strategies in a risk-based manner at Cardiff Intersection Bridge saved £40 million (around $60 million) by cutting 3.5" of required clearance over the standard recommendations [55].
Grid benefits
-
5. For direct electrification of rail, how do you foresee the infrastructure (such as overhead catenary) being built? Who should own and operate the infrastructure?
6. What collaboration with any other entities do you think will be necessary to support the decarbonization of rail transportation?
7. What are the most critical gaps (e.g., with respect to standards, regulations, supply chain, labor) that need to be filled to support acceptance of and markets for alternative rail propulsion technologies?
10. What government actions do you think are necessary to help move the rail sector towards net-zero emissions?
12. What type of workforce challenges are present? Are you aware of any workforce development programs that are relevant to the clean energy transition in the rail sector?
By default, reliable provision of electric traction power requires extension of some degree of transmission capabilities into sparsely populated areas, and DOE should encourage synergies between railroads and utility companies. Like the United States, several other countries such as India, China, and Russia have large expanses of rural land traversed by railroads. Siting transmission lines beside rail lines may well offer opportunities for utility companies to improve reliability to outlying customers, helping to defray some rail electrification cost [56].
In addition, there may be specific legal reasons in the US that favor planning rail electrification and grid expansion together. The US currently suffers a severe lack of transmission that limits the effectiveness of renewable energy projects [57]. Many different separate levels of government have veto power to block or delay a new transmission line [58]. However, US rail projects have long enjoyed special legal privileges that transmission projects currently do not, intended to help them surmount the challenges that long infrastructure projects with diffuse benefits and concentrated negative impacts face [59]. Thus, railway decarbonization may provide a synthesis where utilities, rather than merely leasing rail rights-of-way for transmission purposes, build new transmission along them that in return provide railroads with traction power. Recently announced loan programs would likely facilitate transmission line construction [60].
Moreover, DOE and freight rail companies would be well served to work with US passenger railroads that already have experience with electric traction to develop workforces. Government mandates were key for spurring early 20th century passenger rail electrification in the New York area [61]. Standardization of training programs and operating rules in line with global best practices would increase workforce portability. Generally, railroads build and own the electric traction power infrastructure and operate it in most cases, but there are instances of utility companies managing some or all of the substations.
Conclusion
Decarbonization offers a generational opportunity to transform the American rail network for the better. Traditional direct electrification would provide the best cost-benefit ratio on the overwhelming bulk of the system, in line with experience elsewhere. Moreover, a large body of knowledge on timely and cost-efficient overhead line construction exists for American rail stakeholders to tap into. The Effective Transit Alliance calls on the Department of Energy to encourage adoption of overhead catenary as the proven propulsion solution for a greener future.
Further reading
Free e-book Overhead Electrification for Railways by Garry Keenor https://ocs4rail.com/
National Electrical Manufacturers Association Rail Electrification Coalition response to DOE RFI https://www.nema.org/docs/default-source/council-documents-library/rec-doe-rail-net-zero-emission-propulsion-technologies-comments-february-12-2024-.pdf?sfvrsn=1822979d_3
Official Rail Passenger Association of California and Nevada (RailPAC) response to DOE RFI https://www.railpac.org/wp-content/uploads/2024/01/DOE-rail-RFI-RailPAC-response-2024.01.12.pdf
Download the PDF version of this report
References
https://www.railwaysarchive.co.uk/documents/NR_ElectrificationRUSFinal2009.pdf
https://www.reddit.com/r/trains/comments/vlo2r2/double_stacked_container_train_running_on_western/
https://riagb.org.uk/RIA/Newsroom/Publications%20Folder/Why_Rail_Electrification_Report.aspx
https://www.iea.org/data-and-statistics/data-tools/global-ev-data-explorer
Garry Keenor. Overhead Electrification for Railways, 6th ed. 2021. https://ocs4rail.com/?sdm_process_download=1&download_id=653
https://www.caltrain.com/projects/electrification/project-benefits/caltrain-electrified-service-plan
“Chapter 4: Environmental Health”. Boston Public Health Commission. https://www.bphc.org/healthdata/health-of-boston-report/Documents/7_C4_Env%20Health_16-17_HOB_revised%20Feb%202019.pdf, pp. 15, 17
This is imputed from a breakeven point (benefit-cost ratio = 1) of half-hourly to hourly service with two-car trains.
https://irieen.indianrailways.gov.in/uploads/files/1302522225045-OHE.pdf p. 6
https://onlinepubs.trb.org/Onlinepubs/trr/1985/1023/1023-003.pdf
https://www.railjournal.com/passenger/commuter-rail/sncf-to-renew-paris-rer-line-c-catenary/
https://public.railinc.com/about-railinc/blog/revenue-earning-fleet-continues-slight-growth-q2
https://driveelectric.gov/files/esb-cold-weather-help-sheet.pdf
For example, Siemens just sold 1,200 locomotives to Indian Railways: https://press.siemens.com/global/en/pressrelease/siemens-mobility-awarded-eu3-billion-project-india-largest-locomotive-order-company
https://www.nj.com/news/2021/05/the-advantages-of-electric-trains-without-the-cost-nj-transit-wants-to-test-battery-power.html, https://new.mta.info/press-release/lirr-test-electric-railcars-oyster-bay-branch
See this 2020 BEMU order, $216,000 per linear meter https://www.alstom.com/press-releases-news/2020/2/alstom-signs-first-contract-battery-electric-regional-trains-germany vs. this 2021 order for EMUs at $101,000 per linear meter. https://www.railwaypro.com/wp/alstom-to-supply-32-emus-for-hesse-subnetwork/
https://www.railvolution.net/news/first-coradia-continental-bemu-prepared-for-testing
https://rmi.org/hydrogen-reality-check-distilling-green-hydrogens-water-consumption/
https://www.stuff.co.nz/auckland/local-news/3367763/An-electrifying-start
https://transitmatters.org/reportsfeed/2021/10/20/regional-rail-electrification
https://www.nsar.co.uk/wp-content/uploads/2019/03/RIAECC.pdf
https://www.networkrail.co.uk/wp-content/uploads/2017/09/HOPS-capabilities-and-output.pdf
https://www.thepwi.org/wp-content/uploads/2023/01/PWI-Journal-JAN-2023-WEB.pdf
https://www.railengineer.co.uk/pwi-technical-seminar-electrification-delivering-the-business-case/
https://hsr.ca.gov/wp-content/uploads/docs/programs/eir_memos/Proj_Guidelines_TM3_2_1R01.pdf page 21
https://www.hsr.ca.gov/wp-content/uploads/docs/programs/eir_memos/Proj_Guidelines_TM1_1_21R00.pdf
https://www.hsr.ca.gov/wp-content/uploads/docs/programs/eir_memos/Proj_Guidelines_TM3_2_3R00.pdf PDF page 15–16
https://www.hsr.ca.gov/wp-content/uploads/docs/programs/eir_memos/Proj_Guidelines_TM3_2_3R00.pdf PDF page 18
https://www.railengineer.co.uk/making-electrification-affordable/
https://www.railengineer.co.uk/introducing-surge-arrester-technology/
https://www.hsr.ca.gov/wp-content/uploads/docs/programs/eir_memos/Proj_Guidelines_TM3_2_3R00.pdf PDF page 28
https://www.volts.wtf/p/transmission-fortnight-burying-power#details
https://www.theregreview.org/2021/11/08/pierce-need-to-change-jurisdiction-us-electric-grid/
http://cleanandsecuregrid.org/2017/11/28/north-american-supergrid-permitting-and-regulation/
https://www.energy.gov/articles/doe-announces-825-billion-loans-enhance-electrical-transmission-nationwide / https://www.energy.gov/sites/default/files/2021-04/DOE- LPO_Program_Handout_Transmission-April2021.pdf
Title photograph by Alexey Turenkov and used under Unsplash License.
Autorack train photograph by Lukáš Lehotský used under Unsplash License.
Photograph of ICE on curve by Robert Hale.